The fruit-ripening hormone ethylene
It’s common knowledge that a ripe apple can speed up ripening of green bananas when they’re put together in a bag, and if left too long can rapidly spoil them all. This phenomenon is due to the natural plant hormone ethylene which triggers ripening in fruit, most strongly in those designated as climacteric, and it’s known that it also regulates many other physiological and developmental processes in plants. Exploitation of these effects has led to widespread use in horticulture. It’s difficult to apply in the field because it’s a gas, but this limitation can be overcome by use of an analogue such as ethephon (Ethrel) dissolved in a solution that can then be sprayed onto plants. This is quickly absorbed and redistributed throughout the plant, with subsequent slow release of ethylene.
Examples of the many effects include promotion of fruit ripening in apple, banana, avocado, custard apple, fig, kiwifruit, passion fruit, pear and most stone fruit, de-greening of citrus, synchronising flowering and fruit set in pineapple, faster abscission of flowers and thinning of fruits, breaking seed and bud dormancy in some species (eg peanuts and potatoes), formation of adventitious roots and root hairs, and fruit drop in cherry and walnut. Postharvest fruit can be ripened or de-greened in an enclosed area where released or administered ethylene gas at very low concentrations (ppm or ppb) diffuses into the products, making use of the natural regulatory hormone and minimising concerns over retained artificial additives where fruit might otherwise have been sprayed or dipped. Commercially this means fruit can be picked at an earlier firmer stage when less subject to transport and handling injury and then ripened on demand closer to sale.
Ethylene also finds use in enhancing female sex expression in cucumber, to inhibit self-pollination and increase yield, and to reduce terminal and enhance lateral growth leading to flowering in some species. Post-harvest storage of fruit can be extended by reducing natural ethylene production through reduced temperature and/or increased carbon dioxide levels, or by adding compounds that block its action. Many of these commercially important applications were found by trial and error in the past, and more commonly nowadays through controlled scientific experimentation. What the various findings have demonstrated is that productive outcomes are crucially dependent on a multitude of factors, and consequently the same general management formula can’t simply be applied to all fruit species. The efficacy of ethylene’s effect on enhancing the rate of leaf senescence is illustrated by the analogue 2,4,5-T (Agent Orange) which was notoriously used in the Vietnam war as a defoliant.
The following summary of a comprehensive international study (Chang et al, eLife (2013), 2:e00675, Temporal transcriptional response to ethylene gas drives growth hormone cross-regulation in Arabidopsis) will give you some appreciation of the complexity and co-ordination between hormones in plant biology. Although conducted in the model dicotyledonous plant Arabidopsis related to cabbage and mustard that’s extensively used throughout biology, the general theme is probably a feature of all the higher flowering plants, and many of the genes are largely conserved in fruit crop species. Chang et al found that ethylene activates more than 1300 genes, with 42% being involved in its own signalling pathway and the rest with other hormones. Such cross-talk between hormone actions is not unexpected as plant growth and development requires tight orchestration between hormones having powerful additive, synergistic or antagonist actions. Consider for example the precise regulation of cell wall status with cell growth. Cells are normally under positive turgor pressure to ensure rigidity and movement of diffusible molecules throughout the plant, and this pressure is restrained by the cell wall to prevent rupture. With growth either by cell division or expansion, there has to be exquisite control during insertion of new wall material while maintaining sufficient mechanical strength, a non-trivial feat.
All multicellular organisms, including plants, produce hormones—chemical messengers that are released in one part of an organism but act in another. The binding of hormones to receptor proteins on the surface of target cells activates signal transduction cascades, leading ultimately to changes in the transcription and translation of genes. Ethylene is a gaseous plant hormone that acts at trace levels to stimulate or regulate a variety of processes, including the regulation of plant growth, the ripening of fruit and the shedding of leaves. Plants also produce ethylene in response to wounding, pathogen attack or exposure to environmental stresses, such as extreme temperatures or drought. Although the effects of ethylene on plants are well documented, much less is known about how its functions are controlled and coordinated at the molecular level. Ethylene alters the transcription of DNA into messenger DNA (mRNA) in the plant model organism, Arabidopsis thaliana. Ethylene is known to exert some of its effects via a protein called EIN3, which is a transcription factor that acts as the master regulator of the ethylene signalling pathway. To identify the targets of EIN3, we exposed plants to ethylene and then used a technique called ChIP-Seq to identify those regions of the DNA that EIN3 binds to. At the same time, we used genome-wide mRNA sequencing to determine which genes showed altered transcription. Over the course of 24 hr, ethylene induced four distinct waves of transcription, suggesting that discrete layers of transcriptional control are present. EIN3 binding also controlled a multitude of downstream transcriptional cascades, including a major negative feedback loop. Surprisingly, many of the genes that showed altered expression in response to EIN3 binding were also influenced by hormones other than ethylene. In addition to extending our knowledge of the role of EIN3 in coordinating the effects of ethylene, this study reveals the extensive connectivity between pathways regulated by distinct hormones in plants. The results may also make it easier to identify key players involved in hormone signalling pathways in other plant species.
The following figure summarises their current understanding of how ethylene works:
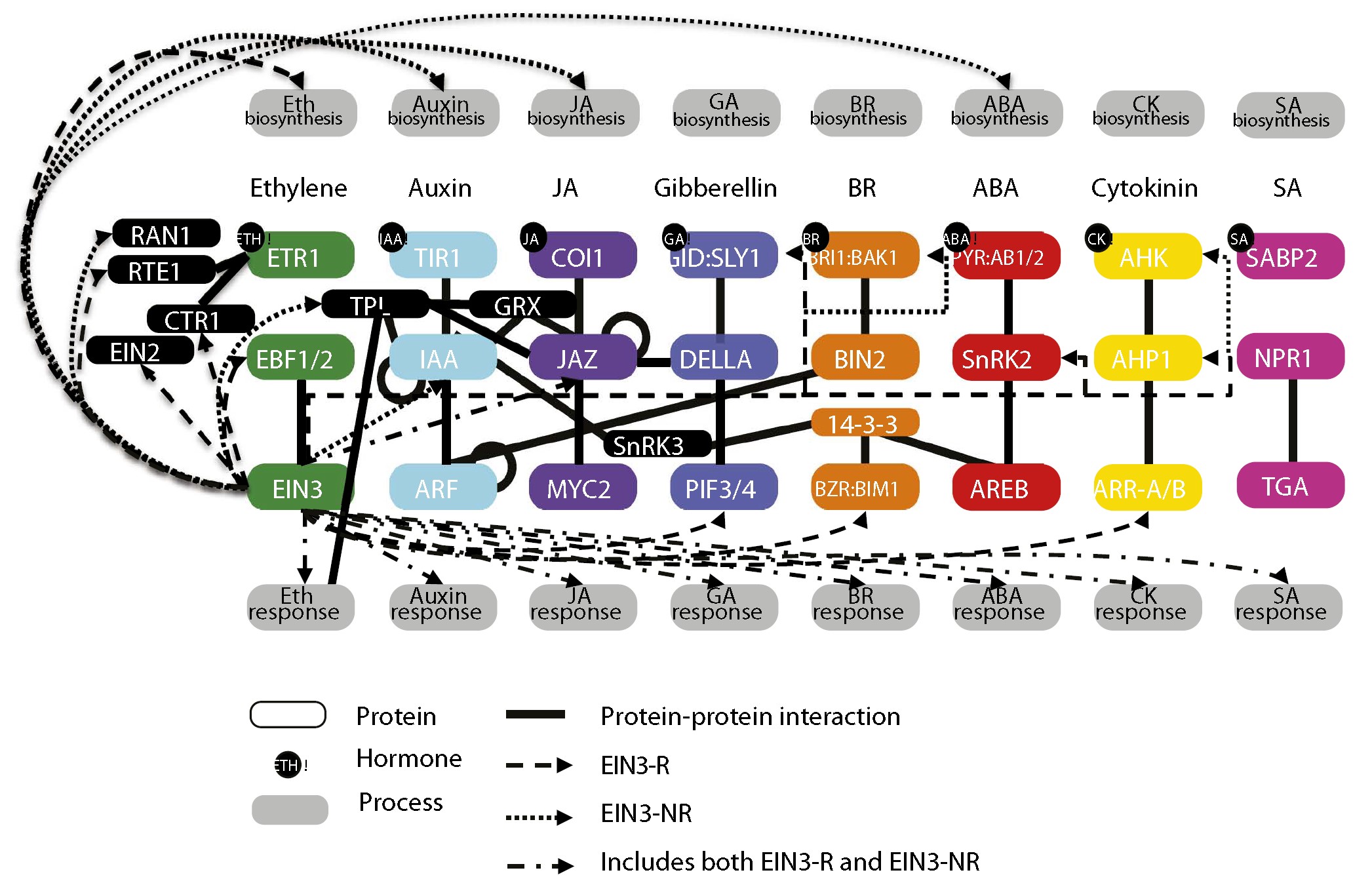
Ethylene signalling pathway and interactions with other hormone actions in Arabidopsis. The top row of grey boxes shows the hormones; Eth - ethylene, auxin (growth), JA - jasmonic acid (defense), GA - gibberellins (plant height and seed germination), brassinosteroids (development), abscisic acid (seed maturation and anti-stress), cytokinins (cell division), and SA – salicylic acid (pathogen defense). The bottom grey row of boxes depicts the effects caused by the respective hormones. Top and bottom rows indicate biosynthetic and response processes respectively. In between these two rows the boxes show other proteins involved in hormone action, including those in black at the left. The small black circles in the second row show the different hormones binding to their receptor proteins. Boxes connected by solid black lines indicate protein-protein interactions.
EIN3 binds to two classes of targets, those where EIN3 is regulated by ethylene (EIN3-R) and those that are independent (EIN3-NR); the dashed and dotted arrows coming from EIN3 indicate the respective co-ordinating effects of these two classes on other proteins and effects. The dotted/dashed arrows show interactions and effects mediated by both EIN3-R and EIN3-NR.
Brian Cull was Director of the Queensland Agricultural Research Station in Nambour and published a revised edition of the book ‘Fruit Growing in Warm Climates’ in 1995. He advised against the naïve use of plant hormones in organic products as they’re very difficult to manage, even when knowledge of the precise hormone mix is known. The problem is that natural plant levels will be augmented or cause a switch in their balance with unpredictable outcomes. Conditions can vary from site to site, year to year, and various species may be affected differently; concentrations and timing are critical. He likened such use to someone firing a shotgun into a chook pen and hoping more eggs will be produced. If there are any positive results it’s likely crop management is astray.
Perusal of the above model of ethylene action reinforces Cull’s prescient advice, as much less was known about the details of hormone actions then. Nevertheless, it was clear to him that plant hormones are very specific, potent and inter-connected regulators of plant activity that can’t be applied across species in a general fashion. In the period since 1995, there have been profound advances in mechanistic understanding with studies such as Chang’s. We can make valuable horticultural use of them in particular circumstances, species and cultivar, hormone concentrations, seasonal timing, degree of fruit maturation etc when we have established the exact procedures to reliably give the desired effects. Hopefully with further advances in the underlying science, even more control will be possible in future with regulation of growth and development, eg in seed germination, fruit ripening, post-harvest storage, response to drought, and insect and pathogen attack.