Absorption of Water-Soluble Nutrients With Foliar Sprays
Foliar nutrient sprays are an increasingly important horticultural management tool world-wide. They can augment supply problems that may exist through normal root uptake processes if soil nutrient profile is inadequate or unbalanced for a healthy crop, or a nutrient is present or applied in sufficient quantities but unavailable because (i) it exists in a tightly complexed form that renders it unavailable for uptake or (ii) adequate amounts may have been applied but were too rapidly washed below the root zone by heavy rainfall. Consequently in these situations with sole reliance on soil supply, general vegetative growth & the additional nutrient demands needed during flowering & fruiting may not be met, leading to sub-optimal fruit quality & yield. Foliar sprays can help address these problems but are best not relied on completely – the mantra is ‘some spraying may be good but more is not necessarily so’. Sprays provide direct nutrient access to foliar tissues after they’ve been absorbed, so only very small quantities are needed with each administration; high concentrations have to be avoided to prevent foliar burn. Low concentrations (usually <1-2%) have the ecological advantage of avoiding wastage that can all too easily occur through soil applications. Excess nutrient application can lead to unnecessary fertiliser & management costs, possible shifts in soil pH & structure, degradation of beneficial microbiota, imbalances in soil nutrient composition that may affect their uptake, & unwanted run-off into aquifers given orchardists often apply too much in an effort to increase yield & profit. Runoff can cause downstream ecological impacts on flora & fauna. A negative consequence of using sprays, alluded to above is, that the amount of nutrients absorbed can be such a small part of what’s needed by the plant, plus even if absorbed, nutrients are often not distributed very far beyond the leaf absorption sites, leading to minimal positive effects throughout the rest of the plant. The importance & promise of foliar nutrient sprays is such that we need to know as much as possible about the process & mechanisms so that effective regimes can be recommended for different species & environments, & we can have confidence in obtaining reliably favourable outcomes that justify the effort & cost.
The following commentary is drawn largely from work by Victoria Fernandes who is an international authority in the field & has published many articles on the topic. How foliar absorption works has proven to be a much more complex question than originally supposed, & teasing out the relative importance of different pathways has been difficult & prone to experimental artefacts. The result is that the phenomenon is still incompletely understood. Much of the earlier research suffered from limitations in experimental techniques available at the time, but in recent years this has improved. The present comments will focus on nutrient absorption through healthy intact leaves, with less on water absorption & only brief comments on how lipid soluble agrochemicals (many pesticides, fungicides, herbicides etc) get absorbed. Above-ground plant vegetative organs can all absorb some nutrients but the pathway that’s most useful for nutrient delivery is through leaves.
The profound challenge with nutrient sprays is variability in response & effectiveness. There are so many factors that can influence this, to the extent that a common saying amongst horticulturalists & researchers is ‘spray & pray’. A similar one is ‘wait for fog, dew or rain and then pray’. Consider some of these factors:
- Species can behave differently to one another. Different canopy regions & developmental stages for the same tree (still juvenile, maturity of plant & leaves) can have variable responses. What worked one year may not the following, & response can also vary throughout a given season.
- Environmental conditions – temperature affects how quickly spray droplets dry out, either before or after deposition on leaves, & nutrients have to be in aqueous solution to be absorbed. Humidity has a comparable effect, with more drying occurring when low & less when high. Rain can wash droplets off leaves. Time of day can impact – if sprayed towards the evening there’s less time for droplets to dry out before dusk.
- Droplet size is important – too small & they become difficult to direct onto the canopy, too big & they may not attach to leaf surfaces & simply run off. If adsorbed on leaves, smaller droplets can give a bigger contact area per volume of spray delivered.
- Plant morphology – eg if leaves are orthotropic compared to distichious, it might be easier to cover (wet) both surfaces with usual spray techniques. Adaxial & abaxial leaf surfaces behave differently, with absorption usually being more effective through the latter. Less mature leaves typically have better absorption.
- Cuticles are biochemically & topologically heterogeneous. Absorption is hampered when they are rough, & better when smooth & thin with many trichomes & veins.
- Nutrient chemical form – eg Mg sulphate, oxide, citrate, nitrate & chelate can all have different effectiveness rankings for absorption of the nutrient of interest, in this case Mg. These rankings may not be the same for different species.
- Deliquescence of nutrient chemicals. This property can vary markedly, even between different salts of the same element. It influences when crystals that have dried on a leaf might redissolve in water absorbed from air, or alternatively come out of solution & start crystallizing.
Leaf surfaces are highly variable. They can be flat or folded, smooth or with varying degrees of roughness due to trichomes & veins that vary in number, the number of stomata with their associated guard cells can vary, & stomata may be encrypted or not depending on the harshness of environmental conditions. Surface microbes, especially those with an irregular exterior (eg fungal mycelia & bacterial flagellae) also add to irregularities. These features mean leaves for some species like aquatics are very wettable & others in arid areas with thicker cuticles to minimise desiccation, more repellent. The interaction of deposited water droplets from foliar sprays, fog, dew or rain on leaves is experimentally measured by the contact angle (CA), the angle from the inside of a droplet where it touches the leaf to the drop periphery or edge. In wettable leaves the CA is <90o & for those that are more repellent it will be >90o. A low CA means the drop will spread out across the leaf surface forming more of a film, resulting in a greater contact area between drop & leaf surface. This larger contact area enhances absorption of water & dissolved nutrients. A high CA means droplets are less spread out & will remain more compact & rounded, with less contact area & less absorption. A rough or folded cuticle can result in a droplet only making contact with the leaf at cuticular peaks, giving little effective contact area between them.
Most synthetic plant nutrients are water soluble, & although almost all are ionised (eg potassium ions) some are not (eg urea and boric acid). The degree of water or lipid solubility of a substance can be described by the hydro-lipid balance (HLB), the ratio of the solubility in aqueous and lipid solvents. Experimentally this can be measured as the water to octanol solubility. Even though plant lipids are much more complex & variable than a simple, single lipid substance like octanol, it nevertheless serves as a reasonable model to make useful predictions. Most mineral nutrients have a high HLB.
The key barrier involved in leaf absorption is the cuticle, a lipid-rich extracellular layer (usually <1mm thick) covering all aerial plant tissues with primary growth capability (leaves, stems, flowers, fruit) to help minimise desiccation; cuticles have even been found on root caps. They also act as a protective barrier against microbial & pest attack, & reduce underlying living cells to excess radiation exposure. They are made up of hydrophobic waxes that may be both epi- &/or intra-cuticular, & (mainly) cutins variably consisting of long chain fatty acid polymers with hydrophilic groups (eg OH & COOH), celluloses, hemicelluloses, pectins, & polysaccharides. Even the waxes can have a few polar groups (2-3%), & specific cuticular components vary across species, plant location & developmental stage. It is now realised that cuticles merge with the underlying epidermal cells, so they are not a totally separate extracellular layer but represent a specialised part of the exterior primary cell wall. The hydrophilic moieties of these polymers can absorb water, so the cuticle expands & contracts depending on humidity (water vapour) & the presence of droplets containing liquid water. The long interior structural cuticular polymer chains are embedded in the waxy lipids (see the Figure) in a way similar to fibre glass, where irregularly oriented glass fibres are enmeshed in a glassy resin. It’s the waxes that mainly confer the lipid-like properties (low HLB ) to cuticles. In the past it was thought most unlikely that water & water-soluble substances could traverse cuticles in any way with such widely differing HLBs. But leaf stomata allow passage of gases (including water vapour) through the stomatal pore & they are regulated by guard cells that can open & shut depending on plant demands of transpiration, photosynthesis, respiration & prevention of desiccation. Historically, these pores, which can be smooth/regular or rough with pegs & extensions, seemed the most obvious pathway for how water soluble nutrients could be absorbed across leaf surfaces. But later it became appreciated that stomata evolved to maintain a clear gaseous pathway across the cuticle for photosynthesis & respiration & to minimise plugging by liquid water, whether containing nutrients or not. The simple idea of liquid water carrying dissolved nutrients across from exterior to interior by bulk liquid flow doesn’t apply because of surface tension – how strongly water molecules are attracted to one another compared to how strongly they are attracted to solid substances, the lipid cuticular lining of the pore in this case. If the former is stronger, then water will form globular-like droplets limited to only the most exterior parts of the pore & leaf surface, resulting in a water repellent leaf. This means the open pore will not be filled all the way through from extra- to the intracellular mileau, & bulk transport of water-dissolved substances from extracellular to the leaf interior cell wall & mesophyll won’t occur. However, with more matching HLBs a droplet or humidity may result in formation of an aqueous film or annulus that lines the pore all the way through while maintaining the pore centre open. Then gaseous transfer can still be maintained and dissolved nutrient will be capable of diffusional movement, exterior to interior, through this thin peripheral aqueous lining. All absorption of spray-delivered nutrients is driven by diffusion, meaning it’s dependent on a concentration gradient.
Surfactant molecules (SA, wetting agents such as non-ionic silicones) form a bridge between lipids & nutrient-containing water – they lower the interfacial tension (reduce the repellency) between water & lipid. They have two parts to them with hydrophilic & lipophilic tails. Across a water lipid interface the hydrophilic end partitions into the water phase & the lipid-like end partitions into the lipid phase, so having part of the molecule in each phase. The interface contrast in HLBs is then less, allowing better interaction instead of repellency. In foliar absorption this promotes development of an aqueous film that can line the pore & greatly assists stomatal passage of dissolved nutrients via this film. The concentration gradient from exterior to interior is usually achieved with sprays containing 1-2% nutrient & the intracellular environment being much less. Absorption of water vapour (without nutrients) can occur through open pores under natural conditions (reverse transpiration), assisting leaves to regain turgor following challenging heat periods where wilting may have occurred. However air humidity usually has to be above 80—90% to provide the concentration gradient (exterior to interior) for this to occur. Appropriate concentrations of SAs can increase the permeability of stomatal pores & cuticles to nutrient uptake by more than 10-fold.
Many studies have shown under the above conditions that stomatal passage of some nutrients can occur, but it’s usually not a large amount throughout each 24hr cycle as most stomata will close at night. Plus, not all stomata are involved in uptake – there are some natural processes, still not understood, that allow some to participate & others not. However, uptake does increase under conditions where more stomata are open. But even at night, or when experimental agents that cause total guard cell closure are applied, substantial uptake still occurs. So we’re left with the concept that water-soluble nutrients can be taken up via another route, namely through the lipid-rich cuticle – seemingly a most unlikely pathway.
An important variable impacting the rate & extent of cuticular uptake is the thickness – the diffusion pathway length. Environmental conditions such as radiation, precipitation, humidity & leaf maturity are also important, & permeabilities in different species can vary by orders of magnitude. The solute can only move across the leaf when in solution, so if drying of the spray occurs before or after deposition, the amount of nutrient taken up into the plant will be compromised. Prevailing humidity affects the droplet drying rate & if low, the spray solution may dry out in air or almost as soon as it’s deposited on the leaf. Temperature will also have an impact. The solubility of nutrients in water will affect how much can be dissolved in the spray solution, & at the upper end is subject to plant toxicity limits. SAs have a similar beneficial effect on cuticular absorption to that for stomata – they reduce aqueous lipid repellency on the leaf surface & allow more ready development of films, enhancing the contact area & absorption. Once nutrients are taken up, they may or may not be distributed to the rest of the plant depending on mobility in the apoplast & leaf phloem. For example, Zn, Mn, Ca & Fe sprays are usually local in their effect with only limited transport out of the sprayed tissues, whereas urea is very mobile & the resultant metabolites are easily transported from mature leaves to sink organs elsewhere. Molecular size is a factor – smaller molecules move faster & further than larger ones. Immature leaves are sink organs & not capable of exporting nutrients until they mature. SAs exhibit phytotoxicity, so determining the right SA and the concentration to use is important. Generally synthetic non-ionic SAs are almost always less harmful than more strongly ionic ones. In addition to widely-used silicones, there are many others, including some organically derived products. Kitchen soaps & household detergents are SAs but they’re almost always strongly ionic (eg sodium lauryl sulphate), alkaline & toxic to plants.
The term deliquescence refers to the ability of substances to take up air-borne moisture if humidity is high or to lose it (dry out) if low. The percent relative humidity (RH) for this phenomenon to occur can vary markedly for different solutes, including individual salts of the same nutrient eg 33% for MgCl2 & 86% for MgSO4. Further complicating the matter, the presence of other dissolved salts can affect the deliquescence relative humidity (DRH). If this is below the RH at a given time, it means the crystallised nutrient salt can take up atmospheric moisture & dissolve in it, allowing leaf uptake to occur. If the DRH is high, it makes it more challenging for dissolution/uptake to occur as the RH has to be higher. You might have noticed the deliquescence phenomenon when a small container of citric acid used for cooking in the kitchen is left open & over time the crystals start sticking together & caking – they’ve taken up moisture because at various times the RH has exceeded the DRH. When overnight temperatures drop & humidity can rise, leaf uptake may be enhanced.
How could it be possible for water soluble nutrients to cross the lipid cuticle? The answer lies in the polymer strands forming the cuticular backbone. These have many polar group s (eg OH, COOH) that can bind water, causing swelling. For example in Citrus x aurantium leaf cuticles, bound water molecules can be up to 7% of the dry cuticular weight, higher in some other species. With sufficient binding, random & often dendritic, some narrow but tortuous chains can span the whole cuticle. The Figure (taken from Fernandes et al, J Expt Botany (2017) 68, 5293) is a stylised representation. If there’s a concentration gradient, which will vary over time in the extracellular region with environmental drying & wetting, water containing nutrients can diffuse across to underlying epidermal cells moving along these chains. They have not been seen under the electron microscope because they’re not permanent pores like stomata, existing only at a molecular scale with usual diameters around 1nm or less, breaking & reforming continuously, not necessarily along the same pathways. This ghostly behaviour depends on random temperature-sensitive molecular motion of the polymer atoms. They have accordingly been termed ‘dynamic aqueous chains’. How many of them are patent at any one time in a leaf depends on the humidity & the availability of water molecules for cuticular absorption. In the Figure, the upper narrow grey band shows epi-cuticular waxes largely devoid of polymers. For two main reasons, it probably represents the major barrier for mineral nutrient absorption – first the HLB is lower because there are fewer polar groups in the lipid molecules (of order 1-2%), & second, the extracellular side of cuticles is usually the least hydrated. The zig-zag over-lapping lines illustrate the structural polymers, & the black dots water molecules that can form clusters temporarily linking up to form chains. The white continuous line is an aqueous pathway where nutrient-containing water can diffuse from extra- to intracellular. There have to be sufficient water molecules (from droplets or humidity) for these continuous pathways to form. Under low humidity conditions, they might only extend a short & insufficient distance towards the extracellular environment, so solute transfer is then not possible. Hydration of the cuticle is usually greater on the intra- compared to extracellular side because of the interior aqueous cellular environment, so the limiting factor for forming continuous cuticle-spanning pathways is almost always on the extracellular side. The extent & rate of osmotically-driven transfer is influenced by cuticle thickness & molecular nutrient size with its associated water-bound shell.
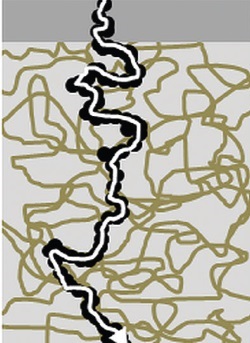
Following the many factors & their implications discussed above, no broad ranging statements can be made about the relative importance of cuticular versus stomatal uptake. But in many instances the cuticular route can dominate given the greater laminal to stomatal area, plus stomatal density is low in some species, some stomata don’t contribute, & they’re not always open throughout the daily 24 hr cycle. One advantage of stomatal uptake is it appears to be less size dependent. Lipid soluble agrochemicals like many fungicides & herbicides are also absorbed via the cuticle, but they do so in a simple osmotically-driven solubility process given more similar HLBs that don’t require passage through dynamic aqueous chains. However their absorption still benefits from appropriate use of SAs as this increases leaf film/contact area.